Eco-friendly and high-resolution reduction of GO biographene papers
We report a novel way for facile writing-in of electrically conductive microscopic patterns (conductivity about 2000 S m-1) by a localized electrochemical reduction with micron-scale resolution of such robust bio-graphene laminated papers with enhanced toughness. Aluminum metal foils have been introduced as an active reducing agent to remove the insulation-inducing epoxide oxygens from graphene oxide. The through-thickness reduction can be well controlled by adjusting the reduction time or reaction parameters. This 3-D electrochemical microstamping writing-in approach can be valuable for future inexpensive, disposable, biodegradable paper with written electrical circuitries integrated into biolelectronic, flexible, and conformal devices.
Gain-Loss Quantum Dot-Polymer Systems Toward Parity-Time Symmetry
Nanoscale particles (quantum dots) have shown tremendous promise in the field of optics due to the tunability of their optical properties. Quantum dots (QDs) show strong potential in optical systems that require strong light amplification (gain) over a range of wavelengths due to their tunable emission and high quantum yields. In addition, the surface of QDs can be functionalized to be soluble in a variety of solvents and compatible with many polymers. Finally, QDs are compatible with many nanoscale deposition techniques and patterning techniques, making them versatile components for a variety of different photonic systems.
This project involves the development of nanoscale films and nanostructures that exhibit high optical gain through the judicial choice of QD surface functionality, polymer matrix, deposition conditions, and pumping schemes. Objectives include the fabrication of ultra-dense QD-polymer and networked-QD films, the physical patterning of these films into nanoscale structures, and the physical and optical characterization of these systems. The in-depth examination of these systems will make it possible to develop systems that satisfy parity-time symmetry (the ultimate goal of this project). Systems that satisfy parity-time symmetry are highly desirable due to their unique manipulation of light, including directional invisibility, directional reflection, and directional energy transfer in coupled laser systems. The successful development of such systems could usher in next generation technologies in the area of photonics.
Materials World Network: Structural Design and Micromechanical Properties of Mechanotransducing Biological Materials
Nature provides numerous examples of model sensing systems which show extraordinary versatility, functionality, sensitivity. Such systems are indispensable for an organism to receive, interpret, and respond to environmental stimuli. In the life of a wandering spider (Cupiennius salei, Figure 1A) perception is dominated by mechanosensory systems, which include tactile hairs air-flow sensing trichobothria and innervated slits embedded in the exoskeleton. More than 3000 so-called slit sensors are present on the body of Cupiennius. The slits are holes embedded in the exocuticle which are generally 1-2 μm wide, ranging from ~10-200 μm in length, and have the ability to detect nanometer-scale strains. Groups of closely-packed slits, known as lyriform organs (examples in Figure 1B & C), work together to detect not only the presence of strain, but also a myriad of strain field properties such as direction, amplitude, or even rate. This collaborative study with the Max Planck Institute of Colloids and Interfaces aims to characterize the fundamental mechanical and structural properties of the spider cuticle of these lyriform organs as well as their surrounding structures.
A spider’s biological vibration filter: micromechanical characteristics of a biomaterial surface.A strain sensing lyriform organ (HS-10) on all legs of a Central American wandering spider (Cupiennius salei) detects courtship, prey, and predator vibrations transmitted by the plant on which it sits. It has been suggested that the viscoelastic properties of a cuticular pad directly adjacent to the sensory organ contribute to the organ’s pronounced high-pass characteristics (Figure 2A). Here, we investigate the micromechanical properties of the cuticular pad biomaterial in search of a deepened understanding of its impact on the vibration sensor´s function. These are considered to be an effective adaptation for the selective detection of signals for frequencies greater than 40 Hz. Using surface force spectroscopy mapping we determine the elastic modulus of the pad surface through a temperature range of 15-40 °C at various loading frequencies. In the glassy state, the elastic modulus was about 100 MPa, while in the rubbery state the elastic modulus decreased to 20 MPa. These data are analyzed according to the principle of time-temperature superposition to construct a master curve that relates mechanical properties, temperature, and stimulus frequencies (Figure 2B). Estimation of loss and storage moduli versus temperature and frequency allowed for the direct comparison with electrophysiology experiments and revealed that the dissipation of energy occurs within a frequency window whose position is controlled by environmental temperatures. Additionally, analysis of sagittal sections of the pad revealed an epicuticle layer which was nearly an order of magnitude thicker than previously measured on other sections of the leg (Figure 3). The thickness of the pad’s epicuticle greatly reduces any contribution from the underlying substructure to the elastic moduluscalculated from AFM probing. The detailed analysis of subsurface morphologies and their role in overall mechanical behavior will be discussed elsewhere.
Structural and compositional characterizationof the spider’s vibrational filter. The detailed information about the stateof the system upon stationary stepwise activation was obtained from in-situ micro-computer tomography (µCT) experiments at synchrotron. Results show that upon stepwise, static loading the pad can be deformed significantly (Figure 4A), suggesting that low frequency mechanical stimuli requires higher deflections in order to achieve a neural response. Furthermore, this result highlights the importance the complex overall and sub-structure of the pad in effective stimulus transmission at low frequency and also in providing mechanical robustness and avoiding damage. In order to better understand the complex structure of the spider pad, small angle x-ray scattering and optical microscopy experiments were performed to determine the chitin fibril alignment and orientation together with those for protein distribution and level of sclerotization (Figure 4B). These results suggest an elaborate organ “design” allowing reliable signal transmittance in wide range of activation frequencies.
Mesoscale Photophysical Properties of Anisotropic Hybrid Nanostructure Assemblies
Hybrid (organic-inorganic) mesoscale assemblies of functionalized anisotropic nanocrystals with tailored shapes and facet-to-facet interactions with conjugated polymers and other materials are investigated in this project. The ability to control light interaction of core-shell, metal-polymer nanostructures is a critical requirement for designing hybrid materials with novel light harvesting and conversion properties for energy materials, catalysts, biosensing, and photonics. Below are some of recent results from this project in collaboration with groups of Prof. El-Sayed and Prof. Reynolds (GA Tech.) and funded by the US Department of Energy.
Coupling of silver nanocubes and gold nano-gratings mediated by a dielectric polymer spacer with controllable nanoscale thickness results in a large enhancement of light absorption (Adv. Funct. Mater. 2014, 24, 6797). Typically, the absorption of plasmonic nanostructures and their assemblies is limited by the narrow spectral bands that arise from wavelength specific resonances. We engineered metal-dielectric-metal nanostructures with broadband absorbing properties in the visible spectral range by combining the plasmonic resonances of different noble metal nanostructures via a hybrid top-down-bottom-up approach. Silver nanocubes and gold nano-gratings were coupled to each other using a dielectric polymer spacer with controllable thickness, resulting in a large multiplicative enhancement of absorption properties across a broad spectral range.
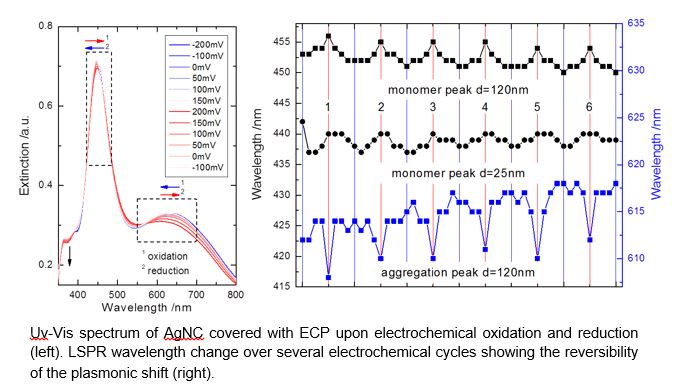
A real-time plasmonic tunability was achieved by embedding silver nanocubes into an electrochromic polymer matrix and applying electrical field (ACS Nano 2014, 8, 6182). Silver nanocubes (AgNC) were assembled on the ITO electrode using LB method and coated with electrochromic polymer (ECP). A significant reversible plasmon peak shift was observed when the nanocube-polymer layer was electrochemically oxidized due to initiated changes in the refractive index of the polymer matrix (as supported by FDTD simulations). The reversibility and repeatability of LSPR shift of AgNC covered with ECP over multiple switching cycles was demonstrated under electrochemical oxidation and reduction of the polymer. The opposite plasmonic resonance shifts were observed for two independent plasmonic modes at the same electrochemical potential and confirmed by FDTD simulations. An increase (or decrease) in the refractive index of ECP coating during electro-oxidation results in a plasmonic red (or blue) shift.
Micromechanical measurements with AFM
For nanomaterials, biological, and polymer composites there is a high demand for characterization of mechanical properties on the micro and nanoscale. We have created software for quantitative acquisition of materials mechanical properties from surface force spectroscopy (SFS) data collected in form AFM indentation data. Nanoindentation is a reliable method of characterization for mechanical properties of materials such as adhesion and elastic modulus with high spatial resolution. These properties depend strongly on operational conditions (time and temperature) for soft polymeric materials and chosen contact mechanics model for the tip-sample interactions. We apply this methodology for mechanical analysis of various soft materials such as polymer films, silicone hydrogel contact lenses, biomaterials (such as spider exocuticle) and silk nanocomposites. In our studies we compare effect of several axisymmetric tip shape models as well as adhesive contact models on calculated elastic properties of these materials. In addition we employed standard linear solid material behavior model combined with force-distance data analysis to extract viscoelastic properties of materials. We have also employed layered models for lamellar systems and evaluated analytical calculations with finite element analysis of experimental data.
Nanostructured Surfaces with Selective Biotraps for Sensing
This project seeks to clarify the fundamental principles guiding the effective utilization of selective binding agents for targeted SERS sensing of important classes of biomolecules such as stress-, performance-, and disease-related biomarkers, and ultimately, to construct sensitive, selective and consistent plasmonic enhanced spectrum biosensors with fingerprinting capability. This far-reaching goal will be advanced in this project through an understanding of the selective binding characteristics of biofunctionalized “hot spots” composed of noble metal nanostructures grafted with active bio-recognition units, such as aptamers or selective peptides (“biotraps”).
Architecture, Assembly, and Emerging Applications of Branched Functional Polyelectrolytes and Poly(ionic liquid)s
Branched polyelectrolytes with ionizable functional groups such as cylindrical brushes, dendritic, hyperbranched, grafted, and star architectures possess complex and unique assembly behavior in solution at surfaces and interfaces as compared with their linear counterparts. The branched polyelectrolytes and poly(ionic liquid)s interact electrostatically with small molecules, linear polyelectrolytes, or other branched polyelectrolytes to form assemblies of hybrid nanoparticles, multilayer thin films, responsive microcapsules, and ion-conductive membranes. The branched structures lead to unconventional assemblies and complex hierarchical structures with responsive properties. Emerging applications of branched polyelectrolytes and poly(ionic liquid)s include energy harvesting and storage, controlled delivery, chemical microreactors, adaptive surfaces, and ion-exchange membranes.
Prediction and Characterization of Light-Matter Phenomena Using Finite-Difference Time-Domain Simulations
Plasmonic materials, namely noble metal nanostructures, allow for the fine control and manipulation of a structure’s electromagnetic fields due to interactions between incident light and free electrons in the material. For instance, light can be confined and guided using plasmonic materials into spatial regimes much smaller than the wavelength of light. While analytical solutions to simple particle geometries, such as spheres, do exist, the light-matter interactions of more complex devices or assemblies is best ascertained by numerical simulation techniques. Surface plasmons are well described by macroscopic electromagnetic theories, such as Maxwell’s equations, if the electron mean free path in the metal is shorter than the plasmon wavelength. This condition is usually met at optical frequencies, meaning plasmonic resonances of metals can be readily identified and modeled by several simulation techniques. Of these different methods, finite-difference time-domain (FDTD) modeling has proven to be one of the more robust and accurate methods for the modeling of SPs. FDTD can be used to design, optimize, and characterize a wide variety of materials relevant to numerous light-matter applications including gain, nonlinear, dispersive, and plasmonic materials.
Ultra-robust graphene oxide reinforced silk fibroin nanocomposites by interphase reinforcement
Reconstituted silk fibroin from Bombyx Mori silkworm silks is an mass-production available source of the excellent biopolymeric material that is strong, transparent, biocompatible, and biodegradable. Ultrathin (<100 nm) films of silk fibroin or its nanocomposites fabricated by either drop casting or spin-assisted layer-by-layer (SA-LbL) techniques are not comparable to natural silk fibers in terms of mechanical properties, mainly because the losing of the hierarchical nanostructures that induced by the biological spinning process. We dramatically reinforced the mechanical properties of the SA-LbL silk fibroin nanomembranes by employing graphene oxide as a strong nanofiller between the layers of silk fibroin molecules.
Completed
Microscopic Analysis of SERS Substrate and Size Distribution of AgNP
Our research goal is focused on understanding the fundamentals of SERS properties and designing a SERS substrate capable of label-free trace detection of biological compounds. We designed SERS substrate using a 3D porous alumina membrane (PAM) decorated with AgNP along the channel walls (Figure 1). Figure 2 shows SEM image of a PAM decorated with 20 nm AgNPs creating hot spots along the pores. The 20 nm AgNP were synthesized reduction of AgNO3 salt by NaBH4. SERS substrate also prepared by the infiltration of 35 nm AgNPs along the PAM nanopores. The utility of photoreduction of AgNO3 salt by NaCitrate produced 35 nm AgNP. Figure 3 shows SEM image of the PAM decorated with 35 nm AgNPs.
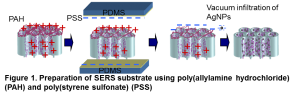
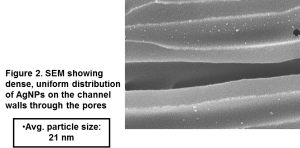
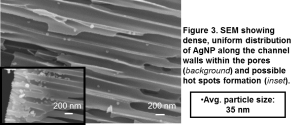
Silver Nanoparticles Synthesis and Characterization
We prepared two different sizes of silver nanoparticles to be used for the formation of hot spots on 3D SERS substrates. The 21 ± 3 nm silver nanoparticles were synthesized reduction of AgNO3 salt by NaBH4. The utility of photoreduction of AgNO3 salt by sodium citrate produced 33 ± 5 nm silver nanoparticles (Figure 4). We collected UV-vis absorption spectra of both particles, which showed an expected red-shift of the 33 ± 5 nm size silver nanoparticle spectrum given the increase in particle size (Figure 5). To characterize the size of silver nanoparticles, AFM height analysis was completed (Figure 6).
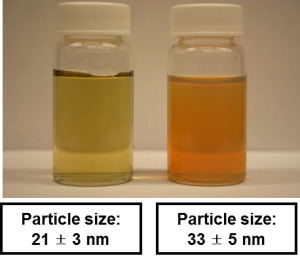
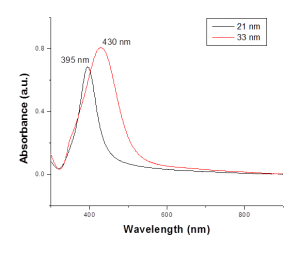
Figure 4. Synthesized silver nanoparticles with different sizes
Figure 5. UV-vis absorption spectra of two different sizes of silver nanoparticles
Figure 6. AFM image of about (a) 21 nm size of silver nanoparticles (z = 100 nm) (b) 33 nm size of silver nanoparticles (z = 200 nm)
Binding of Protein A and IgG to 2D AgNP micropatterned Array
To demonstrate selective binding of protein A to AgNPs, we prepared a 2D AgNP micropatterned array using the capillary transfer lithography method, which is shown in Figure 7 (a). Fluorescein-labeled Protein A (PA*) was deposited on the AgNP micropattern and it was shown that protein A molecules were preferentially adsorbed onto AgNPs Figure 7 (b). Rhodamine-labeled IgG (IgG*) was then deposited on top of the Protein A which was adsorbed on the AgNP micropatterned array. The fluorescence field image Figure 7 (c) shows the selectively binding of IgG molecules to PA. The comparison of AFM phase images between Figure 7 (d) and (e) supports the selective binding of protein A onto AgNPs. The AFM image of the AgNP micropatterned array with IgG will be obtained in future work.
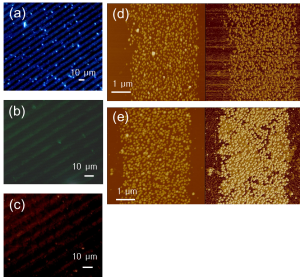
Figure 7. (a) Dark field image of AgNP array (b) fluorescence field image of PA* on AgNP array (c) fluorescence field image of IgG*/PA* on AgNP array, AFM images of AgNP array (d) before and (e) after PA* deposition.
Micropatterned Silver Nanoparticle SERS Substrate
This research was focused on Raman properties and designing a surface enhanced Raman scattering (SERS) substrate capable of label-free, trace detection of biological compounds such as protein A and IgG. We designed a 2D SERS substrate using silver nanoparticles (Figure 1). High resolution AFM images of micropatterned silver nanoparticles were collected to see the difference before and after adsorption of protein A and IgG. The high resolution AFM study of micropatterned silver nanoparticles did not show significant morphological differences in the height image before and after adsorption of protein A, likely due to the small size (Rg ~ 3-5 nm) of these biomolecules. However, the surface roughness of the nanoparticles was shown to change significantly following IgG deposition (Figure 2).
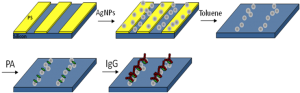
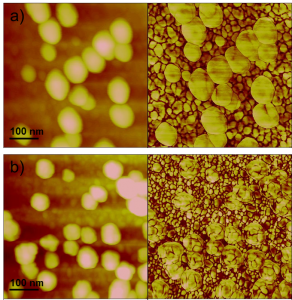
Figure 1. Preparation of 2D micro patterned SERS substrate by capillary transfer lithography using polystyrene pattern
Figure 2. High resolution AFM images of 33 ± 5 nm size of (a) silver nanoparticlle and (c) after protein A and IgG deposition (z = 200 nm)
NSF-DMR
HIGHLY BRANCHED NANOSTRUCTURES AND THEIR ASSEMBLIES
The merit of this research is in the understanding of the fundamental principles of directed assembly of novel highly branched nanostructures: (1) star block copolymers with responsive ionic blocks and (2) highly branched inorganic-organic nanostructures with multiple arm functionalities. We focus on morphology, microstructure, and microdomain morphology of fully organic and hybrid organic-inorganic branched nanostructures at different interfaces as a function of number of arms, chemical composition, external conditions, and surface topology/functionalities by focusing on:
- surface behavior of star block copolymers with emphasis on complex stars with ionic blocks with different molecular architectures on planar and complex templated surfaces with tailored functionalities and topography under different environmental conditions which vary conformation and composition of responsive ionic blocks;
- design and the formation of highly branched inorganic-organic polyhedral oligomeric silsesquioxane nanostructures with variable branching and chemical composition of functionalized arms and their assembling behavior at tailored surfaces and interfaces;
- the understanding of surface behavior and spectral properties of novel “multispectral dots” as inorganic-organic nanostructures with low light scattering and absorption, tunable spectral response, and their directed assembly into nanoengineered materials.
NSF-CBET
NANOPOROUS 3D SUBSTRATES FOR LABEL-FREE TRACE RAMAN DETECTION OF BIOMOLECULES
This NSF award by Biosensing/CBET program supports our work on fundamental Raman scattering properties of nanoporous 3D membranes decorated with aggregates of noble metal nanostructures. Surface enhanced Raman scattering (SERS) substrates designed in this project will be available for ultra-sensitive, label-free probing of trace amount of biomarkers in a biological fluid. Comprehensive analysis of different organic Raman markers and nanoporous structures with variable sizes and shapes is accompanied with electromagnetic modeling to establish design principles for this SERS platform. The knowledge gained from these fundamental studies is utilized to optimize 3D SERS substrates with high enhancement factor for two important classes of biomolecules, immunoglobulin G antibody and prostrate specific antigen. These nanoporous SERS structures possess the advantage of large specific surface area available for the tethering of hot spots with multispectral response; precise control of nanopore diameters and their wall thickness, waveguiding capabilities of transparent cylindrical nanostructures; high robustness and flexibility, and very low cost. The ultimate goal of this project is to achieve robust and reproducible trace detection of femto-mole amount of biomolecules
Raman spectra of the substrate were taken after protein A and after IgG adsorption on the surface. The representative spectra of protein A and IgG are shown in Figure 10. The peak assignments in the SERS spectrum of protein A were proposed based on knowledge of the amino acid sequence of protein A. The spectral peaks of the highest intensity (893, 1291, and 1475 cm-1) can be attributed to lysine, which exists in high concentration in protein A and contributes to its positive charge. As a result of the positive charge of lysine it is thought that this residue on protein A exists in closest proximity to the negatively charged SERS “hot spots” leading to a greater contribution to the overall spectrum of protein A from lysine than other residues present. The characteristic SERS spectrum of IgG was also found following IgG adsorption. Peaks at 1037, 1318, and 1610 cm-1 are indicative of the tryptophan v ring and the peaks at 1318 and 1610 cm-1 also indicate the tyrosine v ring, which are both the characteristic Raman active amino acid sequences of IgG. The characteristic 1610 cm-1 peak indicated above were mapped across the micropatterned sample showing the regions of SERS activity and protein binding (Figure 11).
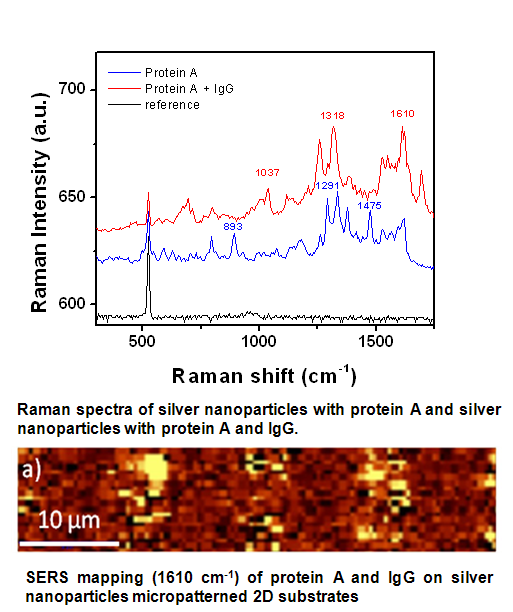
Influence of Pore Diameter on SERS Phenomena
The Raman signal produced from the three different membranes with pore diameters of about 157, 209, and 338 nm showed a clear dependence on the pore diameter of the membranes. The analyte used for these experiments was 10-7 M Rhodamine 6G, which is a standard Raman analyte. The 338 ± 21 nm substrate shows the highest Raman intensity of the three substrates tested when the 1644 cm-1 characteristic peak of Rhodamine 6G is measured (Figure 12). We also collected Raman spectra of 100 ppm of perchlorate from the three different membranes with porediameters about 100, 200, and 300 nm (Figure 13). The PAM with 300 nm pore size showed the better SERS enhancement than other substrates (Figure 14).
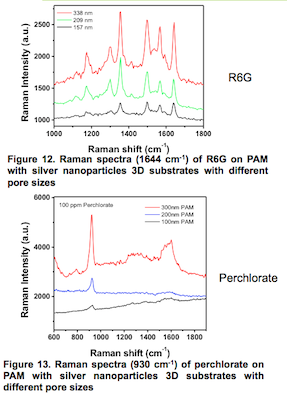
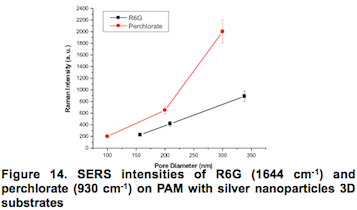
Education –Srikanth Singamaneni, graduated with a PhD and moved to Washington University as an Assistant Professor in January 2010. –Tolecia Clark completed the project in September 2010. –Rajesh Kodiyath started as a post-doc on the project in November 2010. –Sehoon Chang is a senior graduate student, complete his study in May 2010. –Zachary Combs is an NDSEG Fellow and joined the project in August 2009.
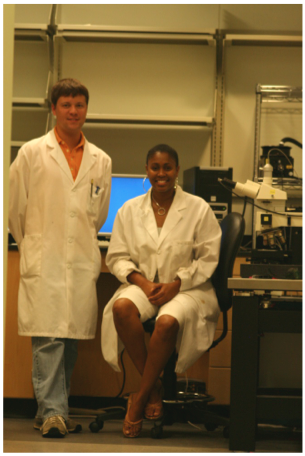
Zachary Combs (left), a graduate student, and Tolecia Clark (center), a post doctoral researcher, from Tsukruk’s group, work on the confocal Raman microscope to analyze novel SERS substrates.
AFOSR-AFRL
The Air Force Center of Excellence on Bio-nano-enabled Inorganic/Organic Nanostructures and Improved Cognition (BIONIC)
Tsukruk, Sandhage, Founding C–directors
The Air Force Center of Excellence (CoE) on Bio-nano-enabled Inorganic/Organic Nanostructures and Improved Cognition (BIONIC) has been established at the Georgia Institute of Technology. Prof. Ken Sandhage (MSE) and Prof. Vladimir Tsukruk (MSE) are co-directors of this center. The center utilizes the expertise and capabilities of integrated teams of Georgia Tech faculty and students (from the Colleges of Engineering and Science), Air Force researchers (from the Materials & Manufacturing Directorate and the Human Effectiveness Directorate of the Air Force Research Laboratory, AFRL), and an Ohio State University (OSU) faculty collaborator.
Tsukruk’s group task: Learn how to design, fabricate, characterize, and model/simulate the performance of inorganic/organic nanocomposites for tunable, adaptive surface structures (Interdisciplinary Research Group 2)
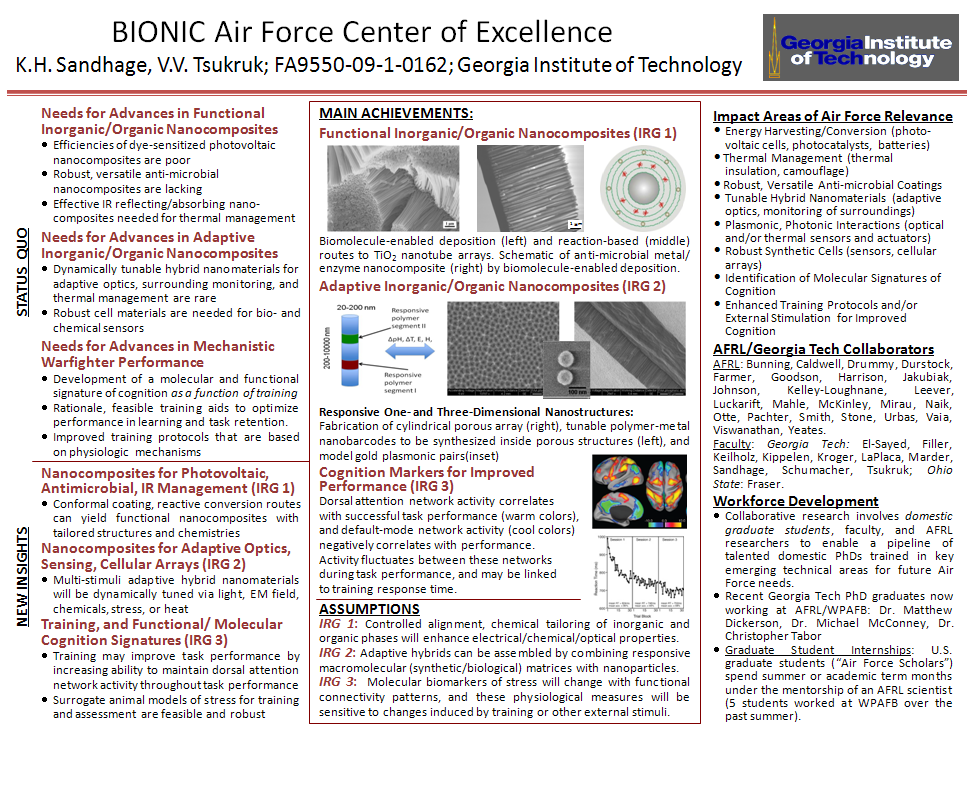
AFOSR
NANOSTRUCTURED INTERFACES FOR ORGANIZED MESOSCOPIC BIOTIC-ABIOTIC MATERIALS
The ultimate goal of this project is to deepen the understanding of the mechanisms and principles of formation of engineered biological-inorganic interfaces and design of organized, integrated, and encapsulated nanostructured materials with complex topologies for prospective biosensing applications. Specifically, our research plan is focused on the fundamental mechanism of surface-mediated formation of nanostructured materials facilitated by selective binding of biomolecules (peptides, proteins, and plasma polymerized polypeptides) gently tethered onto solid substrates via polyelectrolyte complexes with multiple weak ionic interactions and hydrogen bonding. The gained knowledge will be a fundamental basis for prospective ultra-sensing platform from hybrid organized nanomaterials for chemical, optical, and biological applications.
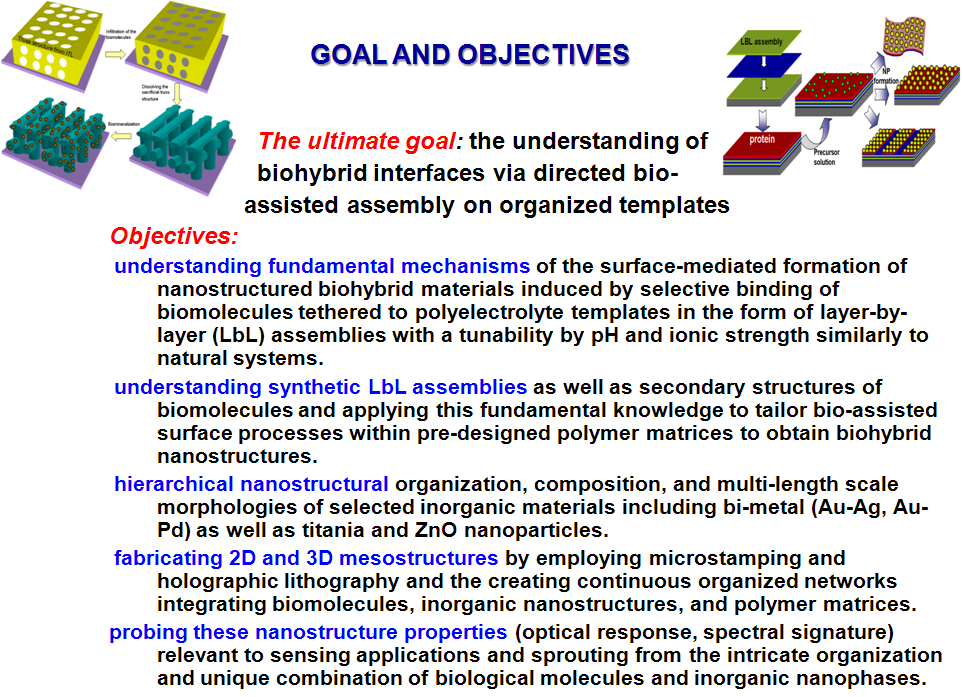
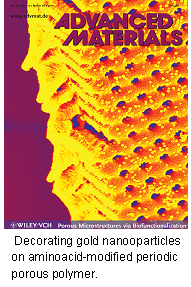
DOE
GUIDED ASSEMBLY OF ANISOTROPIC MICRO-STRUCTURES INTO MESOSCALE HIERARCHIES
Tsukruk, El-Sayed
Combining “dry” and “wet”, hard and soft, organic and inorganic, synthetic and biological materials is a great challenge in the materials science and materials chemistry of soft matter. An understanding of fundamental mechanisms of the formation of hybrid organic-inorganic assemblies and their properties is critical for prospective developments in the fields of bioelectronics, biocatalysts, and biophotonics. To date, no extensive systematic study has been conducted to find “patterns” for the formation of hybrid assemblies from anisotropic microparticles despite the anisotropic building blocks and mesoscopic assemblies are common for biological materials and systems such as cells, vessels, and minerals. In this project, we focus on understanding the principles of formation of hybrid (organic-inorganic) hierarchical mesoscale assemblies from anisotropic microcrystals with tailored shapes and facet-to-facet interactions. In contrast to the current focus on spherical nano- and microparticles, we will be using inorganic anisotropic crystals with well-defined shapes and selectively functionalized facets as building blocks for guided “Lego”-style assembly. This goal is motivated by the fact that despite the wide range of inorganic nano- and microstructures that have been produced by various methods, the guided formation of organized macroscopic and mesoscale materials and structures from anisotropic building blocks has been rarely demonstrated.
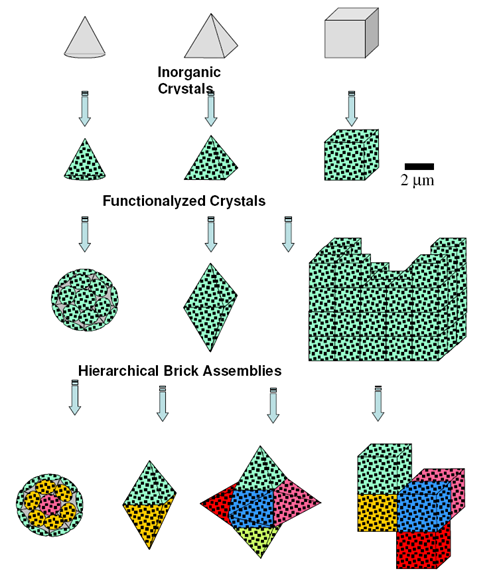
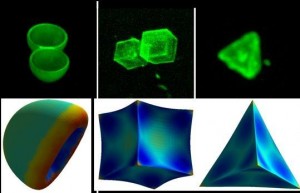
SRC
Fundamentals of Formation and Nanoscale Properties of Carbon Nanotube-Metal Conductor Interfaces.
Tsukruk, Fedorov
This project focuses on the understanding of the fundamental principles of formation of omic contacts at interfaces between multiwalled carbon nanotubes and metal conducts/interconnetcs with emphasis on their non-destructive surface, mechanical, chemical, and electrical property characterization with a sub-micron resolution. The proposed research will facilitate the nanomanufacturing routines by providing comprehensive characterization and offering a novel path for the fabrication of robust CNT-metal interconnects with predictable properties. Understanding the principles of formation of complex CNT-metal interfaces and their properties down to a nanoscale is critical for the implementation of novel nanomanufacturing approaches. Concurrent application of surface interrogating techniques such as AFM, CFM, ChFM, EFM, MFM, and compositionally sensitive techniques such as RRS and SERS will provide invaluable impact. The work suggested here is highly synergetic with approaches proposed in Task 1 and will also directly contribute to Non-destructive Production Measurements by establishing protocols for unambiguous characterization of heterogeneous, carbon-metal interconnects. The proposed research will facilitate the novel nanomanufacturing approaches for the fabrication of high-k dielectric films and highly conductive interconnects suggested in complementary tasks by providing comprehensive characterization tools and offering novel paths for assembly of nanostructured materials.